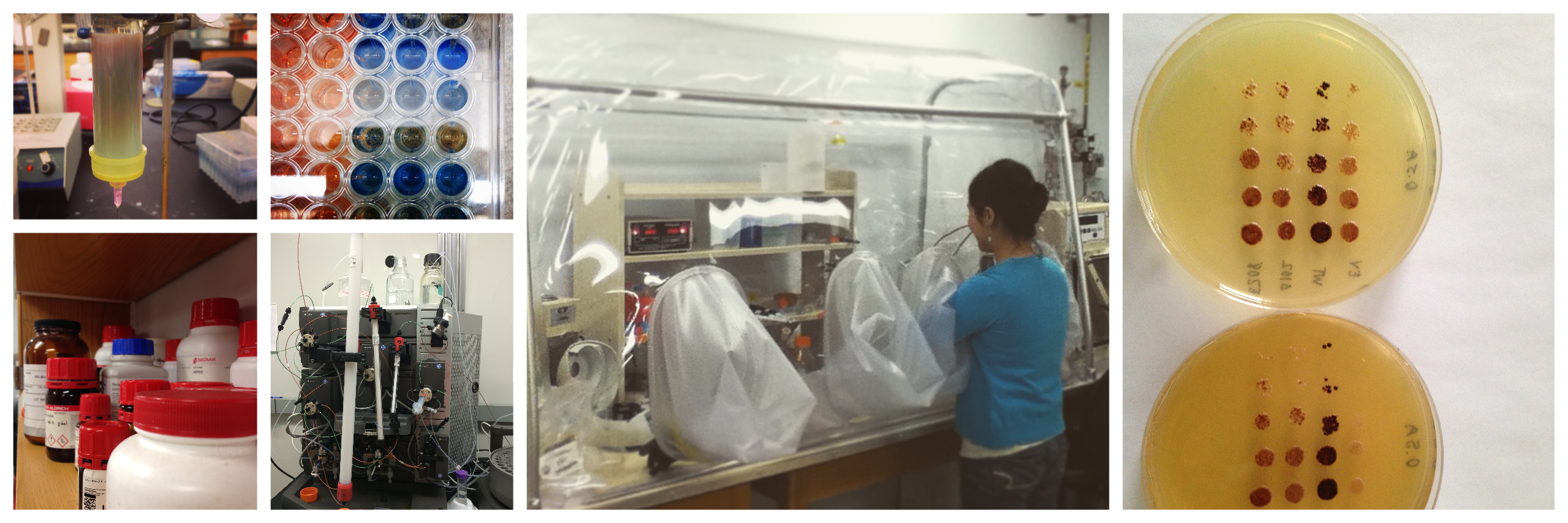
Research
The Perlstein Lab is interested in understanding how iron is utilized by enzymes to catalyze particularly challenging transformations and how iron is delivered into the active sites of metalloproteins in vivo.
Iron is important for cell viability across all domains of life due to the many enzymes which Iron homeostasis in eukaryotic organisms is even more complex because the labile iron pool must not only be divided between the different types of iron cofactors, but it must also be delivered to each subcellular compartment. Both the mitochondria and the nucleus have metalloproteins and there are specific pathways to ensure that the proteins found within these compartments are properly assembled. In the case of iron sulfur cluster cofactors, eukaryotic organisms possess two distinct pathways for FeS cluster biogenesis. There is a mitochondrial pathway that provides clusters to proteins residing inside that compartment, including the proteins of the electron transport chain. There are also more than 25 extramitochondrial enzymes distributed between the cytosol and the nucleus that are FeS proteins. These proteins receive their FeS clusters from the cytosolic iron sulfur cluster assembly pathway. Iron: a critically important and extraordinarily useful cofactor
require iron-containing metallocofactors. There are almost a countless number of mono- and di-iron enzymes, heme-containing enzymes, and iron-sulfur cluster enzymes, just to name a few of the most prevalent types of iron cofactors. Each of these metallocofactors require their own unique biosynthetic pathways that work to divide up the labile iron pool to ensure each type of enzyme is properly assembled. Because excess iron can cause redox stress and a deficit can lead to defects in essential biochemical processes, the cellular concentration of iron must be carefully tuned and controlled.
The Cytosolic Iron Sulfur Cluster Assembly (CIA) pathway biosynthesizes iron sulfur cluster cofactors for extramitochondrial enzymes and it is highly conserved across the eukaryotic kingdom. The CIA pathway is proposed to begin with the assembly of a nascent The CIA targeting complex (CTC) is involved in the last step of the pathway in which apo-targets are identified via a direct protein-protein interaction with the CTC, or through an adaptor mediated interaction. It is unknown if the CTC also donates the FeS cluster directly to the target. In addition, it is not clear how the 20-30 different targets, all differing in their primary and tertiary structures are all recognized by the targeting complex.Overview of the CIA
FeS cluster on the CIA scaffold. In yeast, the CIA scaffold is comprised of two homologous ATPases, called Nbp35 and Cfd1. The Dre2-Tah18 complex is proposed to deliver reducing equivalents required for FeS cluster acquisition by the scaffold. It is currently unknown what small molecules and/or proteins deliver the cluster building blocks. The the direct recipient of Dre2’s reducing equivalents is also not clear. In addition, it is now well understood how the FeS cluster formed by the scaffold gets to the “target”, the apo-enzyme that is the substrate of the CIA pathway. There are at least four proteins are required for the later stages of CIA. In yeast, these proteins called Nar1, Met18, Cia1, and Cia2. Nar1 is distantly related to FeFe hydrogenases and is an FeS protein. But its specific biochemical function during CIA has not been established.
Our lab has developed biophysical assays to monitor nucleotide binding to the CIA scaffold and assays to monitor ATP hydrolysis. We are exploiting these assays to reveal new mechanistic insights into the early steps of the CIA pathway. Investigations into the mechanism of the CIA scaffold
In fungi and animals, the CIA scaffold is comprised of two homologous ATPases, called Nbp35 (NUBP1 in humans) and Cfd1 (NUBP2 in humans). Both proteins contain a CxxC motif near their C-termini which is responsible for binding an FeS cluster which bridges the dimer interface. They also both contain an ATPase site, also found at the dimer interface. The major difference between Nbp35 and Cfd1 is that Nbp35 has an N-terminal FeS cluster binding domain not found in Cfd1. Because these proteins form homocomplexes and heterocomplexes, each quaternary structure of the CIA scaffold binds a different number of FeS clusters: the Cfd1 homodimer can bind one FeS cluster, the Nbp35-Cfd1 heterodimer can bind two FeS clusters, and the Nbp35 homodimer can bind 3 FeS clusters. Because the physiologically relevant form of the scaffold is not known, one of the major focuses of our lab’s work is to try to uncover any differences in activity possessed by each oligomeric state of the scaffold to shed light on whether or not all 3 forms are functional in vivo. In addition, it is not well understood what the role ATP hydrolysis plays during the scaffold reaction cycle.
Our lab is working on finding answers to many long-standing questions about the last step of the CIA pathway. What are the biochemical functions of Nar1, Met18, Cia1 and Cia2? Do all of these subunits participate in target recognition or is this primarily executed by just one subunit, such as Met18? What proteins work to transfer the FeS cluster from the early acting factors to targets? Is Nar1 a FeS cluster carrier? Does the targeting complex bind an FeS cluster? How are targets identified? Do they share a common motif or are there different mechanisms of recognition exploited by different subsets of targets?Cracking the CIA code: unraveling the mystery of target identification
The CIA targeting complex (CTC) is made up of the Met18 (MMS19 in humans), Cia1 (CIAO1 in humans) and Cia2 (MIP18/Fam96B/Cia2b in humans). Previous genetic, biochemical and high throughput proteomic studies have demonstrated that each of these proteins are required for efficient FeS cluster delivery to the CIA targets and that they interact with each other and with the CIA substrates through direct interactions, or through a bridged interaction through the action of an adaptor. Nar1 also binds to the targeting complex.
To uncover the mechanism of CIA, our lab takes an integrative approach. We utilize biophysical assays, spectroscopy, structural biology, enzymology, and yeast genetic analysis. Members of the Perlstein lab obtain a strong foundation in biochemical techniques such as enzyme kinetic analysis through steady state and presteady state approaches. They also learn how to employ new methods, such as bio-layer interferometry and microscale thermophoresis to monitor protein-protein and protein-small molecule interactions. In addition, we routinely incorporate emerging methods from chemical biology, such as unnatural amino acid mutagenesis to incorporate photochemical crosslinking reagents or fluorophores. We also are always looking to expand the reach of our science through active collaborations with other groups in the Boston area and beyond. Our scientific approach