Our Newest Publications in Multi-Scale Materials Architectures
W. Wang, A.B. Resing, K.A. Brown and J.G. Werner. (2024) Electrodeposition of Polymer Networks as Conformal and Uniform Ultrathin Coatings, Advanced Materials. |
Z. Pollard, A. Roshandelpoor, P. Vakili, E.M. Ryan, J. Goldfarb. (2023) Towards tunable polymer foam fabrication: A case study to advance green materials development in limited data scenarios, AIChE Journal.W. | A.B. Resing, C. Fukuda, J.G. Werner. (2023) Architected Low-Tortuosity Electrodes with Tunable Porosity from Nonequilibrium Soft-Matter Processing, Advanced Materials. |
Multi-Scale Sorbents for Efficient Carbon Dioxide Capture and Release (Joerg Werner)
Our goal is to develop a bio-inspired and rationally designed multi-scale material system that removes carbon dioxide from air and makes it available as a purified carbon dioxide at minimum energy demand. A current challenge for the removal of carbon dioxide from air is its relatively low but harmful concentration (0.04%), 2000 and 500 times lower than nitrogen and oxygen, respectively. This necessitates sorbents that are highly selective towards binding carbon dioxide over these other gases. This is achieved by strong binding energies which, however, yield high energy requirements for its subsequent release and sorbent regeneration. Informed by biological systems, our proposed research promises to overcome the selectivity-regeneration conundrum with multi-functional polymers that bind carbon dioxide like a perfectly fitted chemical glove with tunable binding strength. Our molecularly defined capture polymers is realized as thin and uniform coatings on hierarchical porous sorbent architectures to ensure fast air flow and capture of carbon dioxide in the smallest footprint and highest efficiency possible.
Designing Heterogeneous, Hierarchical Porous Materials for Water Filtration (Emily Ryan)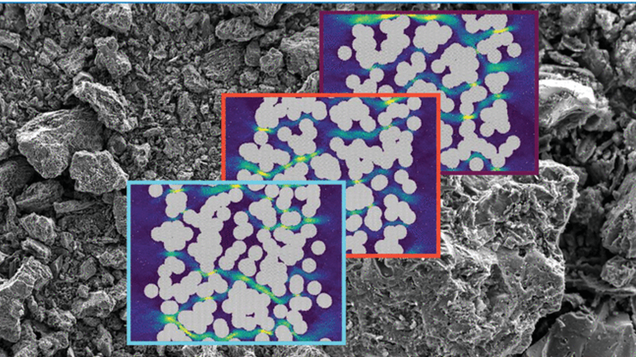
In collaboration with experimentalists at Cornell University, Prof. Ryan’s group is developing an integrated experimental-computational-data science design paradigm for the design of novel materials for sustainability. The large design space of complex materials makes experimental design of new material systems inefficient. Combining experiments with detailed physical models and machine learning methods allows for a more efficient and effective exploration of the design space.
Low-Tortuosity Electrode Architectures to Optimize Energy and Power in Batteries (Joerg Werner)
We explore novel methods to fabricate rationally architected battery electrodes at application relevant scales from the nano- to the micro- and macro-scale. A particular emphasis is on understanding and optimizing the pore architecture to enhance power capabilities (e.g., charging rates) without sacrificing energy density (e.g., driving range for EVs). In recent work from the Werner lab, we demonstrate how straight open micron-sized pores in electrodes can be easily fabricated and tuned using a roll-to-roll compatible bottom-up fabrication method that is material agnostic. We also report initial results on these architectural impacts on battery performance metrics. Find the article here and a “Battery Design” blog post by Anton Resing on his work can be found here.
In a collaborative effort between the Werner, Lubner, and Ryan labs, we are currently advancing our understanding of these effects with operando thermal wave sensing and simulation for spatially probing the lithiation state and unraveling the multi-scale mass transport during fast charge and discharge.
Patterned Anode Interfaces for Improved Battery Performance (Emily Ryan)
Prof. Ryan’s group is exploring the effects of patterned anode surfaces on dendrite nucleation and growth. Engineering interfaces to control mass and charge transport, reactivity, and stability holds significant promise to create longer lasting, higher performing advanced energy technologies.
Advanced Coatings for Energy Devices (Soumendra Basu, Uday Pal, Srikanth Gopalan)
In collaboration with Nissan North America, Profs. Basu, Gopalan and Pal are: i) investigating how to deposit uniform protective spinel coatings on porous interconnects for metal supported fuel cells, and ii) researching spinel compositions for the best coating material. A combination of powder loading, stirring, and AC signal characteristics is being used to successfully deposit uniform spinel coatings on porous substrates with complex geometries. Key properties of undoped and doped Cu-Mn and Mn-Co spinels are being examined to identify optimal coating composition.
Engineering micro/nanostructures on gas-involving electrodes for high-efficiency clean energy production (Chuanhua Duan)
Gas evolving reactions are involved in a variety of electrochemical systems(e.g., batteries, fuel cells and electrolysis cells) and play a critical role in producing clean energy and reducing carbon dioxide emission. Although significant progress has been made to improve the catalyst performance of various gas evolving electrochemical reactions, efficient gas/liquid transport at the electrode, which could also affect the reaction kinetics, have yet to be systematically investigated. In this project, we design and fabricate 3-D hybrid micro/nanostructured surfaces with well-defined dimensions and geometry to enhance gas/liquid transport on the electrode for both gas evolution reactions and gas consumption reactions. The 3-D hybrid micro/nanostructured surfaces utilize patterned microgroove/micropillar arrays and patterned nanowire arrays as well as well-controlled wettability to create separate and efficient transport path for both the liquid and the gas phase while maximizing the electrode-liquid-gas triple phase boundary for the gas involving reactions. The effect of gas/liquid transport on the reaction overpotential and the reaction rate will be thoroughly investigated.
Electrodeposition of Polymer Networks (EPoN) as Ultrathin Coatings on Porous and Non-Planar Electrodes
Chemically precise nanoscale coatings on electrodes, electrocatalyst, or even sorbent materials could enable increased stability (e.g., artificial electrode-electrolyte interphases), tailored chemical specificity, or co-catalytic activity to improve application-relevant performance metrics in batteries, electrolyzers, or carbon capture, to name a few. However, existing fabrication methods struggle to uniformly coat 3-D architectures such porous electrodes with mesoscale feature sizes and high aspect ratios. The Werner lab has developed the Electrodeposition of Polymer Networks (EPoN) as a solution-based processing methods to coat a plethora of functional polymers on porous conductive frameworks using electrochemically mediated crosslinking. We study the foundational mechanisms of our novel polymer electrodeposition paradigm and their use as tunable coatings for multifunctional 3-D materials with large interphase areas for energy and sustainability technologies. Our latest publications on EPoN can be found here and here.